Translate this page into:
Percutaneous thoracic cryoablation of primary lung and pleural tumors: Analysis of long-term experience of feasibility, safety, and recurrence parameters
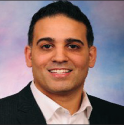
-
Received: ,
Accepted: ,
How to cite this article: Aoun HD, Littrup PJ, Ristic G, Krycia M, Prus M, Baciewicz F, et al. Percutaneous thoracic cryoablation of primary lung and pleural tumors: Analysis of long-term experience of feasibility, safety, and recurrence parameters. Am J Interv Radiol 2020;4:17.
Abstract
Objectives:
The objectives of the study were to report long-term experience with percutaneous thoracic cryoablation, including both malignant pleural and primary pulmonary tumors, assessing factors affecting complications and local recurrence rates.
Material and Methods:
After Institutional Review Board approval and Health Insurance Accountability Act compliance, a retrospective review of 50 patients who underwent 85 CT fluoroscopic-guided percutaneous cryoablation procedures for 116 masses, consisting of non-small cell lung cancer (n = 61), small cell lung cancer (n = 1), mesothelioma (n = 16) and malignant profile fibrous tumors (n = 38) was performed. Tumor size and location (central vs. peripheral) were noted in relation to major vasculature. Hydrodissection and/or esophageal warming balloons were utilized for the protection of crucial structures. Complications were graded according to standardized Common Terminology Criteria for Adverse Events criteria. Patients were followed by CT and/or MRI at 1, 3, 6, 12, 18, and 24 months and yearly, thereafter.
Results:
Mean tumor diameter was 3.3 cm and 3.0 cm for primary lung tumors (PLTs) and malignant pleural tumors (MPTs), respectively. The mean number of cryoprobes utilized was 3.7 per tumor, which produced ablation zones with CT visible ice mean diameters of 5.3 cm. Hydrodissection and/or warming balloon protections were used in 15.3% (n = 13/85). Grade ≥3 complications were limited to tumors >3 cm (8.2% = 7/85; P = 0.0042), but did not appear significant for central location. At a mean follow-up of 17.0 months, overall local tumor recurrence was 6.9% (n = 8/116). Pleural tumors had lower recurrence rates than PLTs (1.8% [n = 1/54] vs. 11.3% [n = 7/62], respectively; P < 0.21). Tumors >3 cm or central location had statistically significant higher recurrence rates, (P = 0.021) and (P = 0.039), respectively. Central recurrences occurred predominantly in tumors >3 cm (20%, n = 5/25).
Conclusion:
With appropriate pre-treatment, evaluation, and procedural criteria, percutaneous cryoablation of both primary lung and pleural tumors is safe and produces very low local recurrence rates, especially for tumors ≤3 cm.
Keywords
Cryoablation
CT guided
Malignant pleural tumors
Percutaneous
Primary lung cancer
INTRODUCTION
Primary lung cancer is the most common cancer and leading cause of cancer death in the United States with approximately 225,000 new cases and 160,000 deaths annually.[1] Treatment of lung cancer is usually driven by stage of disease and patient comorbidities. Minimally invasive effective techniques have become important treatment options for the treatment of lung cancer, especially in non-operable patients and those with high comorbidities.
Surgery, whether minimally invasive or open, has been the gold standard for local treatment of early stage lung cancer (i.e., Stages I–II). Positive surgical margins occur in about 5–15% of patients undergoing a curative procedure for non-small cell lung cancer (NSCLC).[2,3] Complications from lobectomy in a study of 1281 cancer patients undergoing thoracotomy and thoracoscopy were 35% and 26%, respectively.[4] Subsequent local recurrence rates for surgery of primary lung cancer vary between 7% and 12%.[5-8]
More recently, stereotactic body radiotherapy (SBRT) has become more common in the treatment of inoperable lung cancer. Interestingly, local control rates for SBRT are based on annual stratification of recurrences, such as 1 year, 2 years, and 3 years. Recent literature demonstrates 2-year local recurrence rates of approximately 11–12%[9,10] and 16–28% at 3-year follow-up.[10,11] Even with higher treatment doses (~ 80gy), local recurrence rates can be as high as 21–24% after SBRT for central tumors at 2 years.[12,13] Major complication (≥Grade 3) rates after SBRT can be as high as 8% for central tumors.[12]
Percutaneous ablative therapies for primary lung cancer have been predominantly for non-operative patients, using heat-based techniques. Radiofrequency ablation of primary lung tumors (PLTs) has shown local ablation failure between 19% and 38%.[14-16] Even with the increased power and/or intensity of microwave ablation, recurrence rates up to 22–33% have been noted.[17-19] Major complication rates (≥ Grade 3) can be as high as 21% with microwave ablation of lung tumors, many of which are related to prolonged chest tubes for pneumothorax.[20]
Cryoablation is a well-established procedure within various tissues[21-23] including recent data on safety and efficacy of lung tumors.[24-26] Optimum cryoablation techniques stress the production of cytotoxic isotherms (i.e., probe number, probe power, tumor size, etc.) to thoroughly cover all apparent tumor margins.[27] The purpose of this study is to document recurrence rates, complication rates, and imaging outcomes for this large, long-term series of percutaneous cryoablation for PLTs and malignant pleural tumors (MPTs), using consistent procedural parameters. Primary outcome assessments were: (1) Technical feasibility and complications for all PLT and MPT amenable to ablation and (2) imaging outcomes in relation to recurrence rates, tumor size, and organ location. Secondary outcomes included further complication evaluation based on pulmonary function test scores.
MATERIAL AND METHODS
Patients/tumors
All cryoablation procedures were performed between May 2002 and May 2020 under an Institutional Review Board approved protocol for prospective data collection, in compliance with the Health Insurance Portability and Accountability Act. This is a retrospective evaluation of that database (Microsoft Access, Redmond, WA), addressing the primary objectives of cryoablation feasibility and imaging related outcomes for consecutive primary lung and pleural tumors amenable to ablation. Mean patient age was 66.7 years (range 44.5–90.4 years), with 31 masses occurring in men and 85 masses occurring in women.
Inclusion criteria for a primary lung or malignant pleural tumor included any biopsy proven or recurrent neoplasm deemed technically feasible and clinically warranted local treatment as per referring physicians. All the patients were considered medically inoperable because of multiple risk factors and comorbidities or refused surgery. All cases were reviewed and procedures were performed by one of two fellowship trained radiologists with extensive interventional and cross-sectional imaging experience (>25 years and >10 years). Exclusion criteria included patients with underlying coagulopathy, poor pulmonary function (FEV1 and/or DLCO2 <30%), and/or extensive metastatic disease.
Tumor size was limited to standardized treatment methodology with intent of generating thorough cytotoxic ablation temperatures throughout the tumor, preferably in a single ablation, as long as the tumor did not invade major adjacent structures (i.e., mediastinum/heart). All patients had an initial CT or PET/CT within 3 months before cryoablation and each tumor was measured in three dimensions (transverse, anterior-posterior, and craniocaudal) at the level of its largest appearance. Tumor locations were defined as central if the tumor was within 3 cm of the hilum/ mediastinum. Location with respect to lung lobe anatomy (i.e., upper, middle, and lower) was also documented. Patient pulmonary function tests were obtained and reviewed by a dedicated pulmonologist.
Procedures/complications
All lung cryoablation patients received local lidocaine anesthesia (Fresenius Kabi USA, Lake Zurich, IL) and moderate sedation as needed during the procedure per anesthesia staff (e.g., nurse anesthetist or anesthesiologist). Only patients who required esophageal warming balloons for protection underwent general anesthesia (n = 3) while all remaining procedures were performed under conscious sedation. Intravenous antibiotic prophylaxis (e.g., cefazolin 1–2 g, WG Critical Care, Paramus, NJ) was routinely administered before cryoablation, but not continued after. Patients were placed in a lateral or oblique position for comfort (e.g., right decubitus for the left lung tumor access) and secured within a suctioned bean bag (Natus Olympic CAC-PAC, Seattle, WA) for stability on the CT table. No patient was required to be in the prone position during ablation. All procedures were performed under CT fluoroscopic guidance (Siemens Plus 4 with Care Vision fluoroscopy package and more recently Siemens Somatom Sensation 64 slice with Care Vision fluoroscopy package, Erlangen, Germany). Pre-cryoablation CT scans consisted of helical scans without contrast and were reconstructed at 3 mm increments. CT documentation of ice progression during the procedure relied primarily on intermittent CT fluoroscopy. Helical scans, having less quantum mottle, were also obtained for greater clarity at the completion of the first freeze cycle, after the second freeze cycle and post-procedure to evaluate for potential complications.
All of the ablations were performed with a system utilizing the Joule Thompson effect of argon (freezing) and helium (thawing) (Varian Medical Systems, Palo Alto, CA, or Boston Scientific, Marlborough, MA.).[28] Both systems were Food and Drug Administration cleared for lung ablation with variable probe sizes (i.e., 1.5 mm, 1.7 mm, 2.1 mm, or 2.4 mm). Cryoprobe repositioning was avoided by tandemly placing the necessary number of cryoprobes around an initial 20 gauge spinal targeting needle (BD Medical, Franklin Lakes, NJ), or a 17 or 19 gauge trocar needle when a subsequent confirmatory biopsy was needed (Maxcore Biopsy Instrument; C.R. Bard Inc., Covington, Georgia).
For patients requiring biopsy, the trocar needle was placed with its tip at the closest edge of the tumor. One cryoprobe was then placed parallel to the trocar needle according to the treatment plan (below). Biopsies were delayed until the first probe was placed and put to stick freeze to identify tumor margins in case subsequent bleeding after biopsy (i.e., 2–3 cores for histology) potentially obscured tumor location. Remaining cryoprobes were placed according to the plan of the established 1–2 probe placement rule for both PLT and MPT [Figure 1].[22,23,27] Maximum cryoprobe power and surface area were emphasized to select 2.1–2.4 mm cryoprobes when adjacent major vasculature/heat sink (i.e., heart/aorta) was present. No case was performed with a single cryoprobe to avoid non-lethal isotherms.[26] When safely possible, probes were asymmetrically placed closer to adjacent central vasculature to mitigate heat-sink effects.[29]

- A 56-year-old woman with malignant profile pleural tumor who initially presented with chest wall pain. (a) CT unenhanced pre-procedure left lateral decubitus axial image of the chest shows a right upper paramediastinal pleural mass (arrowheads) abutting the trachea (white asterisk) and esophagus containing two esophageal warming balloons (black arrow). (b and c) CT unenhanced intraprocedure left lateral decubitus axial images of the chest showing multiple cryoprobes (white arrows) placed to encompass paramediastinal soft-tissue mass with lethal ice. Hydrodissection fluid at the tip of the hydrodissection needle (black asterisk) and esophageal warming balloons (black arrow) helped protect the tracheal wall and esophagus. (d) CT unenhanced post-procedure left lateral decubitus axial image of the chest shows an ice ball encompassing the right upper lobe paramediastinal mass (arrowheads). Esophageal warming balloons remain in place (black arrow) and mass margin shows early thawing at this point.
The cryoablation procedure consisted of three freeze cycles for all tumors (PLT and MPT) (3 min, ~10 min, and ~5min) separated by two passive thaw cycles (3 min and ~5 min).[30] Initial freeze cycle served to produce a more thermally conductive cloud of interstitial edema and/or hemorrhage around the tumor for better transmission of lethal isotherms beyond tumor margins on subsequent longer freezes.[30] The second freeze cycle was also then better visualized by intermittent CT fluoroscopy showing low-density ice extending ~1 cm beyond prior visible tumor margins, as well as a helical acquisition at approximately 5 min. Where insufficient ice coverage was suspected at the conclusion of the first freeze cycle, an additional cryoprobe would be placed near this tumor margin during the second stick freeze cycle to allow more complete coverage during the second freeze. These extra probes could often be tailored to smaller diameter as needed for coverage completion.
CT images of final ice dimensions were recorded from the post-procedure scans immediately after rapid probe removal (~2 min after thaw), thereby eliminating beam-hardening artifact from the cryoprobes. Protection of adjacent crucial tissues (i.e., esophagus) from cytotoxic freezing temperatures was achieved by either injected saline or transoral warming catheters placed in the esophagus (n = 13) (Varian urethral warming balloons placed in tandem) [Figure 1].[1,31]
Treatment-related major adverse events were noted according to the Common Terminology Criteria for Adverse Events (CTCAE) v5 classification of surgical complications, commonly utilized in thoracic surgery. Complications were also assessed with respect to available pre-procedure pulmonary function test results. Post-procedure pneumothorax incidence and relationship to size and location of tumor as well as probe diameter were documented.
Follow-up
For continuity of care, all post-cryoablation symptomatology and/or complication monitoring were done by the radiology nurse and/or nurse practitioner specified for the ablation program, who was also involved with the cryoablation procedure and scheduling of all follow-up imaging. Follow-up imaging consisted of unenhanced and enhanced CT 1, 3, 6, 12, 18, and 24 months and yearly thereafter [Figure 2]. Follow-up time was noted from the last available CT or PET/ CT, not including the potential additional time from the procedure. The overall ablation zone size[1,32] was measured in three dimensions. Any ablation size increase, or distinct development of asymmetric and/or nodular enhancement, was considered a treatment failure.[1,32] Treatment failures were subclassified as technical failures with recurrence involving the ablation zone or marginal satellite recurrences for those beyond the ablation zone.

- A 46-year-old man who initially presented with cough and underwent wedge resection for a left upper lobe non-small cell lung cancer. (a) CT lung window pre-procedure right lateral decubitus axial image shows left upper lobe pulmonary nodule (arrow) which was biopsy proven to be a post-surgical recurrence. (b) CT intraprocedure right lateral decubitus axial image with intermediate window (W: 1800, L: 200) demonstrates two cryoprobes (arrows) bracketing the left upper lobe nodule. (c) CT lung window post-procedure right lateral decubitus axial image shows the cloud of edema/hemorrhage (arrowheads) surrounding the low-density ice that extends symmetrically beyond the left upper lobe lung nodule margins. (d-f) CT lung window right lateral decubitus axial images at 1-, 3-, and 6-year follow-up, respectively, show progressive resorption of ablation zone with residual linear scar and no significant damage to remaining lung parenchyma. The thorough hypovascular appearance on soft-tissue window (not shown) helped confirm no residual recurrence at year 1 (d), despite the minimal initial resorption at that time.
Statistical analysis
Assessment was limited to observational differences and not intended to power the sample size of the study. All mean value comparisons used the two-tailed Student’s t-test. Frequency comparisons used the Chi-squared test and significance was declared at P < 0.05. R statistical software was utilized to calculate the intraclass correlation coefficient (ICC) among patients.[33] The ICC approached zero and thus the observations were assumed to be independent. Multivariant logistic regression assessment for major complications and local recurrence was also performed based on size and location. Time to local progression (TLP) and overall survival (OS) were only considered for NSCLC patients (n = 45). The Kaplan–Meier survival curve reflecting all-cause mortality and time to progression curves were also generated using the survival package in R statistical software.[33]
RESULTS
Patient/tumors
A total of 50 patients with 116 primary pulmonary masses underwent 85 cryoablation procedures [Table 1]. There were 54 pleural histology origin tumors and 62 parenchymal histology tumors (i.e., NSCL). PFT documentation was available on 70 procedures at the time of data analysis with a mean FEV1 of 72.3% (range: 25–145%) and mean DLCO of 63.5% (range: 27–93%). Biopsy samples (n = 110) showed PLT (n = 62) or MPT (n = 49). The MPT biopsy samples included mesothelioma (n = 11) and malignant profile fibrous tumors (MPFTs) (n = 38). One patient with MPFT of the pleura had 36 masses cryoablated over approximately 4 years, some of which arose in the contralateral lung. A second patient also had MPFT of pleura and two masses ablated in separate settings.
Demographic | Mean (range) | ≤3 cm | > 3cm | Peripheral | Central | |||||
---|---|---|---|---|---|---|---|---|---|---|
Mean (range) | Mean (range) | Mean (range) | Mean (range) | |||||||
Gender (M/F) | 20/30 | |||||||||
Age (years) | 66.7 (44.5–90.4) | |||||||||
FEV1 (%) | 72.3 (25–145) | 73.9 (32–145) | 70.8 (25–95) | 74.0 (47–108) | 71.3 (25–145) | |||||
DLCO (%) | 63.5 (27–93) | 65.2 (27–93) | 60.9 (33–83) | 64.4 (32–93) | 62.4 (27–85) | |||||
Tumor demographics | ||||||||||
Tumor histology | n | Tumor size (cm) mean (range) | n | Tumor size (cm) mean (range) | n | Tumor size (cm) mean (range) | n | Tumor size (cm) mean (range) | n | Tumor size (cm) mean (range) |
NSCLC | 61 | 3.3 (0.7–8.7) | 34 | 2.1 (0.7–3.0) | 27 | 4.8 (3.1–8.7) | 30 | 2.8 (0.7–8.7) | 31 | 3.7 (1.0–8.2) |
SCLC | 1 | 1.7 (1.7) | 1 | 1.7 | 0 | 0 | 1 | 1.7 (1.7) | 0 | 0 |
Mesothelioma | 16 | 3.6 (1.8–8.0) | 8 | 2.2 (1.8–2.8) | 8 | 5.0 (3.6–8.0) | 14 | 3.1 (1.8–5.1) | 2 | 6.8 (5.5–8) |
Fibrous tumor | 38 | 2.8 (1.0–9.5) | 29 | 2.1 (1.0–3.0) | 9 | 4.9 (3.1–9.5) | 24 | 2.2 (1–3.6) | 14 | 3.6 (1.1–9.5) |
Location | ||||||||||
Upper lobe | 44 | 2.6 (0.7–8.2) | 35 | 1.9 (0.7–3.0) | 9 | 5.2 (3.2–8.2) | 26 | 2.0 (0.7–6.4) | 18 | 3.7 (1.1–8.2) |
Middle lobe | 20 | 3.8 (1.2–8.7) | 8 | 2.4 (1.2–3.0) | 12 | 4.7 (3.1–8.7) | 11 | 4.2 (2.2–8.7) | 9 | 3.3 (1.2–5.4) |
Lower lobe | 50 | 3.3 (1.0–9.5) | 29 | 2.2 (1.0–3.0) | 21 | 4.9 (3.1–9.5) | 30 | 2.3 (1.5–4.9) | 20 | 4.4 (1.0–9.5) |
Procedure demographics | # of procedures |
Tumor size (cm) mean (range) |
Number of probes (per tumor) |
Probe/tumor ratio | ||||||
Probe size (mm) | ||||||||||
1.5 | 4 | 1.9 (1.1–2.9) | 3.2 (2–4) | 1.7 | ||||||
1.7 | 15 | 2.5 (0.9–6.6) | 3.2 (2–5) | 1.28 | ||||||
2.4 | 66 | 3.3 (1.2–9.5) | 3.8 (2–11) | 1.2 |
Patient, tumor, and procedure demographics for 116 masses in 50 patients and 85 procedures. Values in parenthesis are ranges
Of the malignant pulmonary intraparenchymal lesions, 61 were related to NSCLC (48 adenocarcinoma and 13 squamous cell) and 1 was related to small cell cancer. The patient with small cell lung cancer had stable disease for 2 years and interval growth of two small adjacent pulmonary nodules (0.5 cm and 0.8 cm) who presented for cryoablation of oligometastases. Six tumors had a history of previous NSCLC with post-radiation or post-surgical recurrent suspicious masses which underwent rebiopsy at time of cryoablation and came back as normal lung parenchyma, likely related to sampling artifact. PET positivity and/or CT growth were still considered clinically suspicious for recurrence by consensus. Sixteen tumors were related to biopsy-proven mesothelioma.
Thirteen patients had multiple masses ablated and 14 procedures (8 patients) had multiple masses (up to 4 masses) ablated in one setting. Overall average mass size for parenchymal origin tumors (i.e., NSCL and small cell) and pleural origin tumors (i.e., mesothelioma and malignant fibrous tumor) was 3.3 (range: 0.7–8.7) and 3.0 (range: 1.0– 9.5), respectively. The MPFT patient who underwent ablation of 36 masses in 20 procedures had an average tumor size of 2.4 cm (range 1.0–4.8 cm). There were 43 masses >3 cm and 73 masses ≤3 cm, with no significant size difference noted between upper lobe (n = 44), mid (n = 20), and lower (n = 52) lobe location (P > 0.05) [Table 1]. Tumor size and location were noted in relation to complication and recurrences as follows.
Procedure/complications
An average of 3.6 and 4.0 cryoprobes was used per tumor for the PLT and MPT, respectively, which corresponds to approximately one probe (2.4 mm) per centimeter of tumor. Table 1 shows that 78% of procedures (n = 66/85) used 2.4 mm cryoprobes for all tumor sizes, while the <2 mm diameter cryoprobes were generally used in smaller tumors. The mean immediate post-procedure ablation zone (ice ball) size was 5.1 cm (range: 2.4–10.9 cm) and 5.59 cm (range: 2.7–11.0 cm) for PLT and MPT, respectively. Hydrodissection (n = 10) and/or warming catheter balloon (n = 3) protection of adjacent vital structures (i.e., esophagus) were performed in 15.3% of (n = 13/85) procedures.
Total major (Grade ≥3) complications for this series were 8.2% (n = 7/85), which included all procedure related and unrelated incidents within 1 month post-ablation. There were 8.9% (n = 5/56) and 6.9% (n = 2/29) major complications for PLT and MPT, respectively. No statistical significance of location (central vs. peripheral and lobe distribution) was noted for major complications (P = 0.77) [Table 2]. Procedures for tumors >3 cm accounted for all major complications, compared with procedures for tumors ≤3 cm (P = 0.0042) [Table 2]. Multivariant logistic regression in procedures with one tumor (n = 62) demonstrated significant increase in major complications for procedures with larger tumors (OR 1.48, 95% CI: 1.01–2.18, P < 0.05). However, tumor location (central vs. peripheral) did not significantly affect multivariant complication rates (OR 1.61, 95% CI: 0.25–10.3, P = 0.62).
Twenty-one procedures (n = 21/85; 24.7%) had intraprocedure or immediate post-procedure pneumothorax of which 9 (n = 9/85; 10.6%) required small (8–10F) catheter aspiration (CTCAE grade 2). Pneumothorax rate was not statistically greater in central tumors (P = 0.66) or tumors >3 cm (P = 0.12). One patient required prolonged chest tube >2 days secondary to a bronchopleural fistula and required surgical management (Grade 3). No greater pneumothorax rate was seen for patients with limited PFT (FEV1<50%) (P = 0.89). Incidence of pneumothorax was greater in the patients where 1.7 mm (n = 7/15; 47%) probes were utilized versus 2.4 mm probes (n = 14/66; 21%) (P = 0.042). No pneumothoraces were present in procedures which utilized the 1.5 mm probes (n = 4).
There were five deaths in this series, all in the PLT group, three of which were attributed to the procedure and two that were not attributed and occurred within 30 days of procedure. Two patients (6.8 cm and 3.4 cm tumors) had myocardial infarctions, one of which was not related to the procedure, within 30 days (day 1 and day 6, respectively) which resulted in deaths. One patient had respiratory compromise post-procedure with an 8.5 cm tumor with 45% DCLO and 47% FEV1 pre-procedure, whose family discontinued ventilatory supportive care on day 1 post-procedure. Another patient with a 4.8 cm tumor with 40% DLCO and 25% FEV1 had autopsy proven inadvertent intrapulmonary 16F chest tube placement in recovery for a presumed pneumothorax that led to rapid respiratory compromise after placement. The fifth patient had a CT confirmed pulmonary embolism within 30 days of the procedure.
Follow-up/recurrences
The median and mean follow-up was 10.7 months and 17.0 months, respectively, with a range of 1 month–7.1 years. Of the 12 masses (10.5%) which had over 3-year follow-up, one patient had ≥5-year follow-up. The local recurrence rate was 6.9% (n = 8/116), including both technical failures (n = 2/116; 1.7%) and peripheral local recurrences (n = 6/116; 5.2%).
Eight tumors (6.9%) demonstrated local recurrence by imaging criteria of which 3 (n = 3/8; 37.5%) were confirmed with rebiopsy. Seven (11.3%) tumors demonstrated local recurrence for PLT and 1 (1.8%) demonstrated local recurrence for MPT. All recurrences were along the margin of the ablation zone [Figure 3]. Tumors >3 cm or central location had significantly higher recurrence rates, (P = 0.021) and (P = 0.039), respectively [Table 2]. Recurrence rates were significantly lower for MPT (1.8%=1/54) versus PLT (11.3% = 7/62; P = 0.045). Multivariant logistic regression analysis (n = 115) demonstrated increased local recurrence for tumors of increasing size (OR 1.40, 95%CI: 1.02–1.93, P = 0.037). The effect of tumor location (central or peripheral) on multivariant logistic regression for local recurrence was not significant (OR: 4.90, 95%CI: 0.945–25.4, P = 0.0585).

- A 45-year-old woman who had an incidental lung mass found on CT for shortness of breath. (a) CT lung window pre-procedure left lateral decubitus axial image shows biopsy-proven right lower lobe lung cancer (arrow). (b) CT intermediate window (W: 1800, L: 200) intraprocedure left lateral decubitus axial image demonstrates multiple cryoprobes (arrows) in the right lower lobe mass during early freeze. The intermediate window utilized here allows for better visualization of the probes, ribs, and mass at one time. (c) CT lung window post-procedure left lateral decubitus axial image shows a cloud of edema/hemorrhage surrounding right lower lobe mass (arrowheads), but focal gap in the cloud is <1 cm from tumor (arrow). (d and e) CT lung and soft-tissue window, respectively, 3-month follow-up left lateral decubitus axial images show focal nodular enhancing recurrence along anterolateral margin (white arrow) of the remaining avascular ablation zone (arrowheads). (f) CT soft-tissue window intraprocedure left lateral decubitus axial image of recurrence reablation shows multiple probes extending anterior through ablation zone and beyond nodular recurrence (arrow). (g) CT lung window post-procedure left lateral decubitus axial image of recurrence ablation now shows cryoprobe track extending through nodular recurrence (black arrow). (h) Contrast-enhanced CT soft-tissue window left lateral decubitus axial image at 1 year follow-up of recurrence ablation shows thorough avascular ablation zone (arrowheads).
Recurrences total (%) | Recurrences | Major complications | |||||||
---|---|---|---|---|---|---|---|---|---|
Primary lung tumors %) | Pleural origin tumors (%) | Grade | Total (%) | Primary lung tumors (%) | Pleural origin tumors (%) | ||||
3 | 4 | 5 | |||||||
Size | |||||||||
≤3 | 2.7 (2/73) | 5.6 (2/36) | 0.0 (0/37) | 0 (0/44) | 0 (0/30) | 0 (0/14) | |||
>3 | 14.0 (6/43) | 19.2 (5/26) | 5.9 (1/17) | 1 | 1 | 5 | 17.1 (7/41) | 19.2 (5/26) | 13.3 (2/15) |
Location | |||||||||
Central | 12.8 (6/47) | 16.1 (5/31) | 6.3 (1/16) | 1 | 3 | 9.1 (4/44) | 6.7 (2/30) | 14.3 (2/14) | |
Peripheral | 2.9 (2/69) | 6.5 (2/31) | 0.0 (0/38) | 1 | 2 | 7.3 (3/41) | 11.5 (3/26) | 0 (0/15) | |
Probe size | |||||||||
1.5 | 0 (0/4) | 0 (0/3) | 0 (0/3) | 0 (0/4) | 0 (0/3) | 0 (0/1) | |||
1.7 | 5.9 (1/17) | 7.7 (1/13) | 0.0 (0/4) | 1 | 6.7 (1/15) | 7.7 (1/13) | 0 (0/2) | ||
2.4 | 7.4 (7/95) | 11.5 (6/52) | 2.3 (1/43) | 1 | 1 | 4 | 9.1 (6/66) | 10.0 (4/40) | 7.7 (2/26) |
Total | 6.9 (8/116) | 11.3 (7/62) | 1.8 (1/54) | 1 | 1 | 5 | 8.2 (7/85) | 8.9 (5/56) | 6.9 (2/29) |
Local recurrences and major complications (CTCAEv5) were assessed based on size, location, tumor origin (pleural vs. intraparenchymal), and probe size. Tumors >3 cm, central location, and non-pleural origin had statistically higher rates (P=0.021, P=0.039, and P=0.045, respectively). No statistical significance between probe size (2.4 vs. 1.5 and/or 1.7) and recurrence rates (P=0.67). Major complications were statistically significant for tumors >3 cm (P=0.0042)
Table 2 also shows that nearly all central recurrences occurred in tumors >3 cm, resulting in recurrence rate up to 20% (5/25). Probe size (1.5 mm and 1.7 mm vs. 2.4 mm) and number were not related to recurrence rates (P = 0.67). Assessment of OS and TLP curves for only the patients with NSCLC demonstrated that they were more likely to die from other causes rather than local recurrence at the primary lung cancer ablation site [Figure 4].

- Graph of time to local progression and overall survival. Death from other causes is much more likely than local recurrence.
DISCUSSION
Treating PLT in patients who have multiple comorbidities or have contraindications to surgery can be challenging. CT-guided visualization of cryozone margins and understanding the lethal isotherms in treating PLT produced low recurrence rates, especially in tumors ≤3 cm (2.7%).[27] The overall recurrences included technical failures as well as marginal satellite recurrences. There was a statistical trend for greater recurrences for tumors >3 cm (14.0%) or in a central location (12.8%), which emphasizes the need for greater lethal isotherms to counter heat sink and extend beyond tumor margins. However, multivariant assessment of recurrences relative to central location was not significant and was more influenced by the size of tumors (>3 cm). Local recurrence rates are significantly lower compared with heat-based ablations (22–38%) and even for tumors >3 cm.[14,15,17,18] Although SBRT has had good short-term control rates, tumor recurrence increases at 2- and 3-year follow-up and can be as high as 28%.[9-11]
The low procedure pain and excellent soft-tissue healing parameters of cryoablation allow the unique inclusion of more MPT in this series.[23] Indeed, 46.6% (54/116) of tumors undergoing cryoablation were MPT (MPFT or mesothelioma). While 69% (36/52) of these originated in the patient with a MPFT of the pleura, it also highlights the broad tissue type cytotoxicity of the treatment planning protocol and consistent probe placement technique. While a single case may appear anecdotal, it speaks to the patient procedure satisfaction. The patient chose to return for 20 repeated outpatient procedures over 4 years while maintaining 100% performance status, which would not have been possible for surgical management. Considering that MPFT of the pleura has 25% recurrence rate,[34] such chronic maintenance with a minimally invasive, low morbidity outpatient procedure offers a valuable treatment option.
This long-term series of thoracic cryoablation over 18 years is, therefore, a unique combination of PLT and MPT, which allowed overall procedure assessment for nearly all primary tumors arising in and around the thorax. Complication and recurrence rates were related to more standard risk parameters of tumor size or central location. MPT had less recurrences, but was likely related to ease of cytotoxic ice coverage in locations with less adjacent heat-sink vasculature, rather than differential cellular sensitivity to lethal isotherms. From a procedure perspective, one must be cognizant with technical cryoablation parameters that need to be altered for thoracic tumors that may involve lung parenchyma, pleura, or chest wall.
This cryoablation protocol was predicated on covering all tumor margins with cytotoxic tissue temperatures delivered by sufficient cryoprobe density, which provided some insight into whether probe size or number had associated impact on complications or recurrences. The pneumothorax data present a counterintuitive greater rate for the 1.7 mm probes than the larger 2.4 mm probes, although, the probe to tumor ratio was slightly greater in the 1.7 mm group (1.28 vs. 1.15, respectively). This was likely arbitrary and possibly related to extent of centrilobular emphysema which we did not grade for this study.
Despite the non-invasive aspect of SBRT, major complication rates can be as high as 8% for central tumors.[12] More powerful and uniform heat-based ablations (e.g., microwave) have major complication rates of up to 21%.[20] There were no major complications in this study for tumors <3 cm in both the peripheral and central locations. Complications were significantly greater in tumors >3 cm (17.1%), but not related to central locations. Although there were five early deaths in this series, two were unrelated to the procedure and two may have been preventable. All of the attributable deaths occurred in tumors greater than 4 cm. Most of the patients were not surgical candidates due to moderate comorbidities, however, the immediate post-procedural mortality rate of 3.5% (n = 3/85) was comparable to surgical lobectomy mortality which can be as high as 3.1%.[35] Strict limits on tumor size and pulmonary function status can limit mortality in these inoperable patients with moderate comorbidities.
There were limitations to this study. This was a retrospective review of a large series without randomization or a control arm (i.e., surgery or observation). Yet, this would be quite difficult since many of our patients were non-surgical candidates with multiple comorbidities. A second limitation of our study is the limited power and comparison of complications and recurrence rates relative to probe size utilized for procedures. Another limitation is that the extent of underlying lung disease (i.e., emphysema or fibrosis) was not assessed in this study. Finally, the patients in this study had moderate comorbidities and were inoperable, so there was a bias toward treating sicker patients rather than all comers.
CONCLUSION
Percutaneous CT-guided cryoablation is a highly efficacious alternative for the treatment of primary thoracic tumors originating from the lung and pleura for both central and peripheral tumors <3 cm. For tumors >3 cm, patient pulmonary function status and other comorbidities should be considered before management, including appropriate procedural safety considerations for skin, nerve, and esophagus.
Acknowledgments
We would like to thank Dr. Salah Abdelhadi, MD and Barbara Adam MSN, APN for their help and contributions toward the preparation manuscript.
Declaration of patient consent
Institutional Review Board permission obtained for the study.
Financial support and sponsorship
Nil.
Conflicts of interest
There are no conflicts of interest.
References
- CT-guided percutaneous cryotherapy of renal masses. J Vasc Interv Radiol. 2007;18:383-92.
- [CrossRef] [PubMed] [Google Scholar]
- Microscopic residual disease after resection for lung cancer: A multifaceted but poor factor of prognosis. Ann Thorac Surg. 2010;89:870-5.
- [CrossRef] [PubMed] [Google Scholar]
- Residual disease at the bronchial stump after curative resection for lung cancer. Eur J Cardiothorac Surg. 2007;32:29-34.
- [CrossRef] [PubMed] [Google Scholar]
- Thoracoscopic lobectomy is associated with lower morbidity than open lobectomy: A propensity-matched analysis from the STS database. J Thorac Cardiovasc Surg. 2010;139:366-78.
- [CrossRef] [PubMed] [Google Scholar]
- Tumor recurrence after complete resection for non-small cell lung cancer. Ann Thorac Surg. 2012;93:1813-20. discussion 1820-1
- [CrossRef] [PubMed] [Google Scholar]
- Survival after recurrent nonsmall-cell lung cancer after complete pulmonary resection. Ann Thorac Surg. 2007;83:409-17. discussioin 417-8
- [CrossRef] [PubMed] [Google Scholar]
- Local failure after complete resection of N0-1 non-small cell lung cancer. Lung Cancer. 2011;71:156-65.
- [CrossRef] [PubMed] [Google Scholar]
- Post-recurrence survival in completely resected Stage I non-small cell lung cancer with local recurrence. Thorax. 2009;64:192-6.
- [CrossRef] [PubMed] [Google Scholar]
- Central versus peripheral tumor location: Influence on survival, local control, and toxicity following stereotactic body radiotherapy for primary non-small-cell lung cancer. J Thorac Oncol. 2015;10:832-7.
- [CrossRef] [PubMed] [Google Scholar]
- Survival outcome after stereotactic body radiation therapy and surgery for Stage I non-small cell lung cancer: A meta-analysis. Int J Radiat Oncol Biol Phys. 2014;90:603-11.
- [CrossRef] [PubMed] [Google Scholar]
- Outcomes after stereotactic body radiotherapy for lung tumors, with emphasis on comparison of primary lung cancer and metastatic lung tumors. BMC Cancer. 2014;14:464.
- [CrossRef] [PubMed] [Google Scholar]
- Local control and toxicity in a large cohort of central lung tumors treated with stereotactic body radiation therapy. Int J Radiat Oncol Biol Phys. 2014;90:1168-76.
- [CrossRef] [PubMed] [Google Scholar]
- Stereotactic body radiotherapy for centrally located early-stage non-small cell lung cancer or lung metastases from the RSSearch(®) patient registry. Radiat Oncol. 2015;10:113.
- [CrossRef] [PubMed] [Google Scholar]
- Percutaneous radiofrequency ablation of clinical Stage I non-small cell lung cancer. J Thorac Cardiovasc Surg. 2011;142:24-30.
- [CrossRef] [PubMed] [Google Scholar]
- Primary non-small cell lung cancer: Review of frequency, location, and time of recurrence after radiofrequency ablation. Radiology. 2010;254:301-7.
- [CrossRef] [PubMed] [Google Scholar]
- Radiofrequency ablation of Stage IA non-small cell lung cancer in patients ineligible for surgery: Results of a prospective multicenter Phase II trial. J Cardiothorac Surg. 2018;13:91.
- [CrossRef] [PubMed] [Google Scholar]
- Microwave ablation of lung malignancies: Effectiveness, CT findings, and safety in 50 patients. Radiology. 2008;247:871-9.
- [CrossRef] [PubMed] [Google Scholar]
- Percutaneous microwave ablation of Stage I medically inoperable non-small cell lung cancer: Clinical evaluation of 47 cases. J Surg Oncol. 2014;110:758-63.
- [CrossRef] [PubMed] [Google Scholar]
- Local efficacy and survival after microwave ablation of lung tumors: A retrospective study in 183 patients. J Vasc Interv Radiol. 2016;27:1806-14.
- [CrossRef] [PubMed] [Google Scholar]
- Major complications after lung microwave ablation: A single-center experience on 204 sessions. Ann Thorac Surg. 2014;98:243-8.
- [CrossRef] [PubMed] [Google Scholar]
- Percutaneous cryoablation of renal tumours: Outcomes from 171 tumours in 147 patients. BJU Int. 2013;112:758-65.
- [CrossRef] [PubMed] [Google Scholar]
- Percutaneous cryoablation of hepatic tumors: Long-term experience of a large U.S. series. Abdom Radiol (NY). 2016;41:767-80.
- [CrossRef] [PubMed] [Google Scholar]
- Soft-tissue cryoablation in diffuse locations: Feasibility and intermediate term outcomes. J Vasc Interv Radiol. 2013;24:1817-25.
- [CrossRef] [PubMed] [Google Scholar]
- Percutaneous cryoablation for the treatment of medically inoperable Stage I non-small cell lung cancer. PLoS One. 2012;7:e33223.
- [CrossRef] [PubMed] [Google Scholar]
- Evaluating cryoablation of metastatic lung tumors in patients-safety and efficacy: The eclipse trial-interim analysis at 1 year. J Thorac Oncol. 2015;10:1468-74.
- [CrossRef] [PubMed] [Google Scholar]
- Five-year survival after cryoablation of Stage I non-small cell lung cancer in medically inoperable patients. J Vasc Interv Radiol. 2015;26:312-9.
- [CrossRef] [PubMed] [Google Scholar]
- Lethal isotherms of cryoablation in a phantom study: Effects of heat load, probe size, and number. J Vasc Interv Radiol. 2009;20:1343-51.
- [CrossRef] [PubMed] [Google Scholar]
- Cryoablation: Mechanism of action and devices. J Vasc Interv Radiol. 2010;21(Suppl 8):S187-91.
- [CrossRef] [PubMed] [Google Scholar]
- Perivascular and intralesional tissue necrosis after hepatic cryoablation: Results in a porcine model. Surgery. 1997;122:742-7.
- [CrossRef] [Google Scholar]
- Optimizing the protocol for pulmonary cryoablation: A comparison of a dual-and triple-freeze protocol. Cardiovasc Intervent Radiol. 2010;33:1180-5.
- [CrossRef] [PubMed] [Google Scholar]
- Thermal protection during percutaneous thermal ablation of renal cell carcinoma. J Vasc Interv Radiol. 2004;15:753-8.
- [CrossRef] [PubMed] [Google Scholar]
- Imaging features following thermal ablation of lung malignancies. Semin Intervent Radiol. 2013;30:157-68.
- [CrossRef] [PubMed] [Google Scholar]
- R: A Language and Environment for Statistical Computing Vienna: R Foundation for Statistical Computing; 2013.
- [Google Scholar]
- Solitary fibrous tumors of the pleura: A single center experience at National Cancer Center, China. Thorac Cancer. 2018;9:1763-9.
- [CrossRef] [PubMed] [Google Scholar]
- Thirty-day mortality after lobectomy in elderly patients eligible for lung cancer screening. Ann Thorac Surg. 2016;101:541-6.
- [CrossRef] [PubMed] [Google Scholar]